Biological and bioinspired photonics
Understanding the optical properties of natural systems can not only shine light on their biological relevance but also serve as an inspiration for the fabrication of sustainable materials. These two aspects of my research are detailed in the following.
Bioinspired white materials
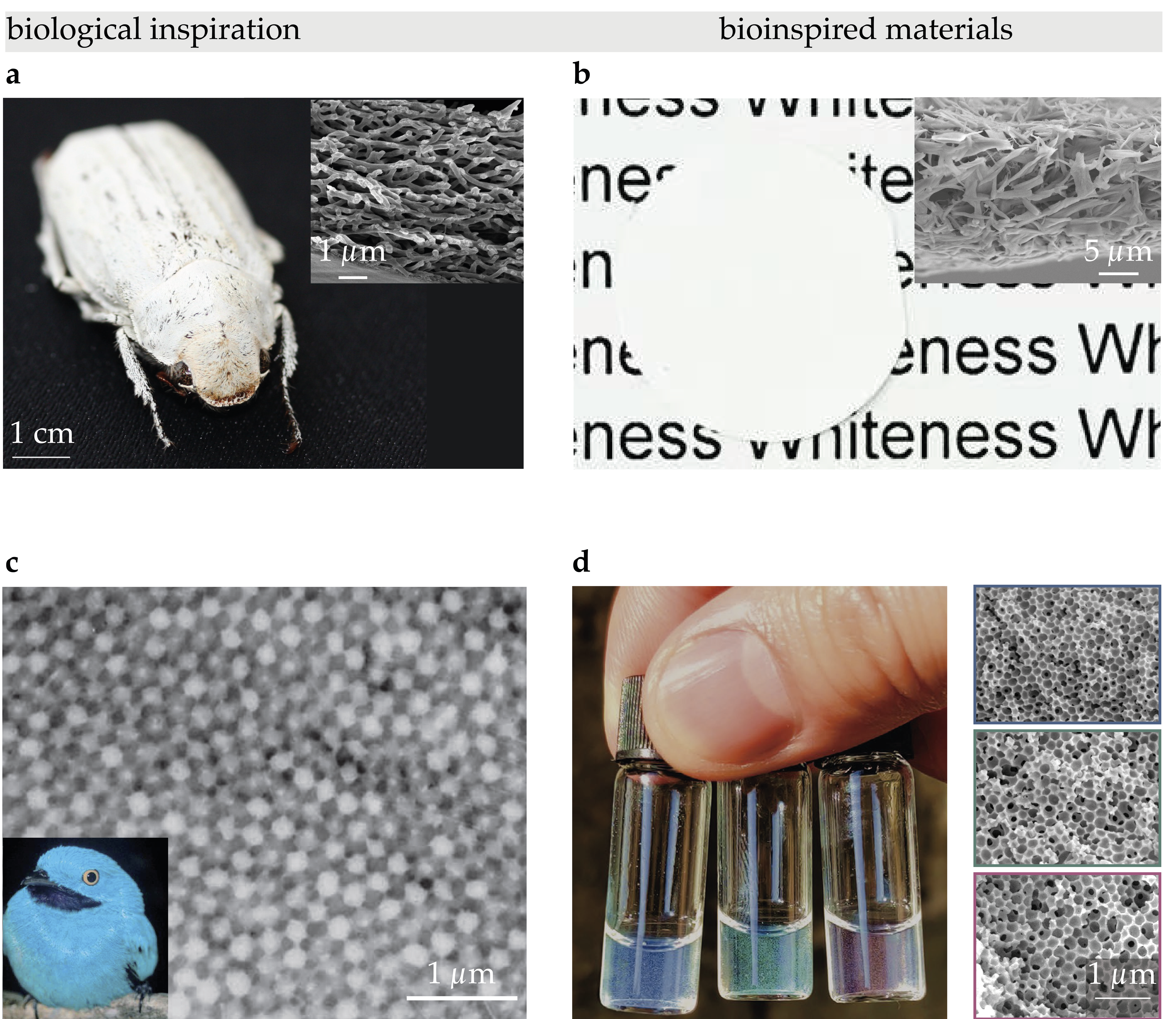
Nature provides a striking example of scattering optimisation with the beetle genus Cyphochilus. This insect shows a white appearance obtained via the chitin network found in the scales covering its exoskeleton (Figure 1a). I demonstrated that it is the anisotropic nature of this network that gives rise to such a high scattering efficiency (more details here). Following this biological inspiration, I worked in close collaboration with materials scientists and chemists to translate this knowledge into the fabrication of bioinspired white materials solely made of biopolymers. I was part of four projects exploiting different techniques—ranging from the fabrication of anisotropic colloids to chemical methods to obtain cellulose fibers of the desired size—to obtain materials with a whiteness comparable to the Cyphochilus beetle. In one of these studies, we developed a protocol to fabricate cellulose-based microparticles with a controlled size and aspect ratio. Moreover, their scattering response was further optimised by controlling the spatial arrange- ment of these particles in a disordered network with a controlled degree of alignment and filling fraction. The technology developed in this work was patented and it was instrumental to the birth of a lab spin-off named Impossible Materials (now Seprify), that has as its main goal the mass production of such bionspired white materials to give a sustainable and biocompatible alternative to titanium-dioxide pigments (Figure 1b). This latter have been shown to be toxic and have been recently banned from the european market. Therefore, the presented bioinspired white pigments could fill this important gap that finds a lot of application not only for paints but also for food colourants and other daily goods.
in short: I studied light propagation in disordered microscale architectures in biological systems. This research translated into the fabrication of bioinspired white pigments, incorporating insights from nature into advanced material development.
main collaborators:
- Hendrik Hölscher (KIT, Germany);
- Orlando Rojas (Aalto University, Finland);
- Stefan Bon (Warwick University, UK).
Angle-independent pigments
As discussed in the Fundamental Optics page of this website, the fabrication of bioinspired pigments with tunable and angular-independent colours relies on a delicate balance between the single-scatterer and ensemble contribution to scattering. We exploited this knowledge to fabricate polymeric materials achieving matte structural colouration across the entire visible spectrum (Figure 1d). These materials were obtained via a self-assembly process that we called “controlled micellisation”. In short, by limiting water permeation within an emulsified toluene-in-water droplet, we achieved porous structures with controlled pore sizes and consequent coloration. Our work will be instrumental in assisting the fabrication of angle-independent pigments for applications ranging from colorants in automotive paints and exterior coatings to use as pixels in reflective displays.
in short: I investigated light propagation in microscale architectures with varying degrees of order. Leveraging this knowledge, we fabricated of bioinspired pigments with tunable and angular-independent colours.
Role of disorder on the optical properties of biological systems
Beyond the applied interests mentioned in the previous sections, thanks to the collaboration with world-leading teams of biologists and plant scientists, the numerical and experimental tools I developed to study short-range ordered systems demonstrated to be of tremendous help to understand the role of structural disorder in the functionalities of biological systems. Specifically, I exploited numerical tools to study how the microstructures present in the eyes of mantis shrimp larvae affect their visual perception. Furthermore, I applied similar tools to investigate the hereditary nature of colouration in beetles and to deduce the structural organisation of bacteria colonies from their optical response. Figure 2a shows the iridescent response arising from the complex three-dimensional organisation (Figure 2b-d) of the Flavobacterium IR1. Through numerical analysis of the angle-resolved optical responses of bacterial colonies, we demonstrated the capability to estimate inter-cellular spacing and diameters with sub-10 nm precision, surpassing the resolution limitations faced by traditional electron microscopy due to sample preparation distortions. Moreover, by correlating the optical response with the degree of disorder through computational models, we also gained detailed insights into the bacterial structural organisation. Importantly, I also collaborate to apply similar techniques to derive from the angular-resolved response of cholesteric films of cellulose nanocrystals information of their self-assembly pathway.
Beyond animals and microorganisms, I also studied the biological functions of the optical properties of certain plant and fruit species. More specifically, my work contributed to demonstrating the photoprotective function of the golden sheen in Tradescantia pallida leaves and the signalling function of the blue colour in Viburnum tinus fruits. In particular, we demonstrated that the origin of the colour observed in V. tinus fruits (Figure 2e) originates from multilayered assembly of lipid inclusions. Numerical simulations further demonstrated that this colouration is influenced by both on the degree of disorder present in the multilayer and also in the lipid content, suggesting it as a reliable indicator of the fruit’s nutritional content.
The capability of designing microstructures made of bio-compatible materials described in the previous sections could also serve to improve light delivery in biological systems. To prove this idea, I collaborated on the design and optical characterisation of coral-inspired biomimetic approaches to optimise the photosynthesis of microalgal colonies. Specifically, we embedded microalgae in a 3D-printed hydrogel that emulated coral morphology, resulting in a marked increase in photosynthetic efficiency due to improved light delivery. In particular, we incorporated in our bioinspired device cellulose nanocrystals, which act as scatterers and whose concentration can be controlled to optimise light delivery to the algae. Figure 2g-i demonstrate the biocompatibility of the 3D-printed scaffold, showing that the microalgae grow by forming dense aggregates. This work showcases the potential of a biohybrid approach in photosynthetic problems.
in short: I exploited experimental and numerical tools to enhance our understanding of disorder’s role in the optical responses of diverse biological systems.

main collaborators:
- Beverley Glover (University of Cambridge, UK);
- Daniel Wangpraseurt (UC San Diego, USA);
- Miranda Sinnott-Armstrong (University of Colorado-Boulder, USA)
- Rox Middleton (University of Bristol, UK)